Efficient by Design Through Innovative Cooling Technology
Tower Tech is the leading manufacturer of products that effectively bridge the gap between sustainability and energy efficiency. Tower Tech offers the highest quality products with patented technology providing innovative solutions.
Industries Served
-
Tower Tech is the leader for modular cooling towers in industrial applications. Our towers are the strongest and most efficient on the market and have FM Approvals for fire, storm and seismic ratings. We specialize in serving:
- Chemicals & Plastics
- Oil & Gas
- Manufacturing & Food
- Automotive & EV Battery
- Semiconductors & Data Centers
- Sustainability & Emerging Markets
-
Tower Tech is the leader for factory-built cooling towers in HVAC and Comfort Cooling applications. Our towers are the most efficient towers on the market and have the lowest total cost of ownership. We specialize in serving:
- Healthcare
- Education
- Hospitality
- Government & Military
- Commercial Real Estate
-
Tower Tech is the leader for applications where redundancy and energy efficiency are paramount. Our cooling towers can remain up-and-running even if a fan or other modular component goes down. When is comes to flexibility Tower Tech towers provide maximum utility to power plants and utilities providers.
-
Tower Tech is the leader for applications that are seasonal or necessitate a short term cooling tower solution. Our cooling towers are modular and because they are factory built they can be easily transported and installed. Our technology can get your application or facility's cooling back on-line in the shortest amount of time.
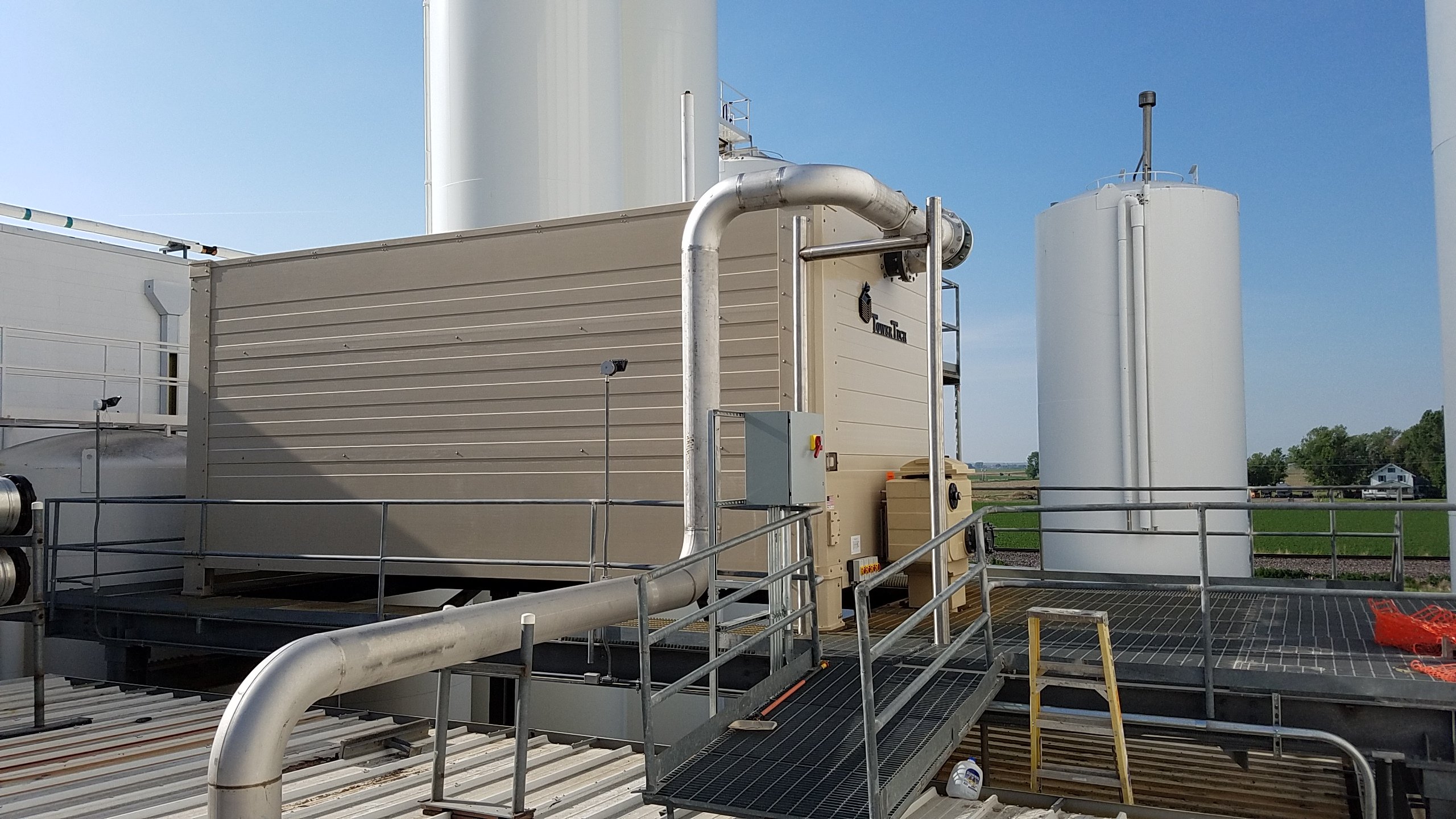
Tower Tech is the leader for modular cooling towers in industrial applications. Our towers are the strongest and most efficient on the market and have FM Approvals for fire, storm and seismic ratings. We specialize in serving:
- Chemicals & Plastics
- Oil & Gas
- Manufacturing & Food
- Automotive & EV Battery
- Semiconductors & Data Centers
- Sustainability & Emerging Markets
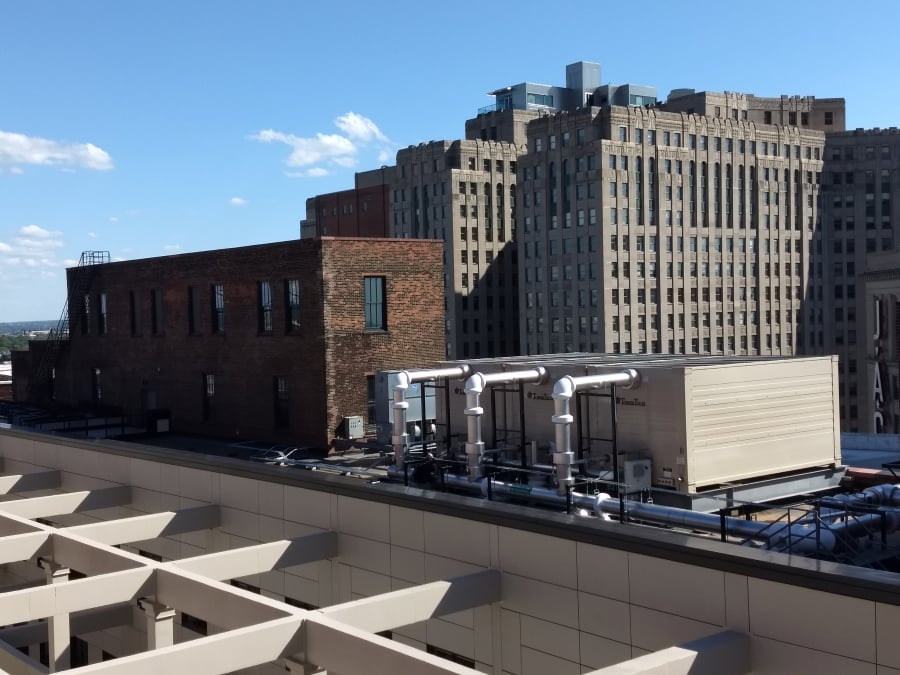
Tower Tech is the leader for factory-built cooling towers in HVAC and Comfort Cooling applications. Our towers are the most efficient towers on the market and have the lowest total cost of ownership. We specialize in serving:
- Healthcare
- Education
- Hospitality
- Government & Military
- Commercial Real Estate
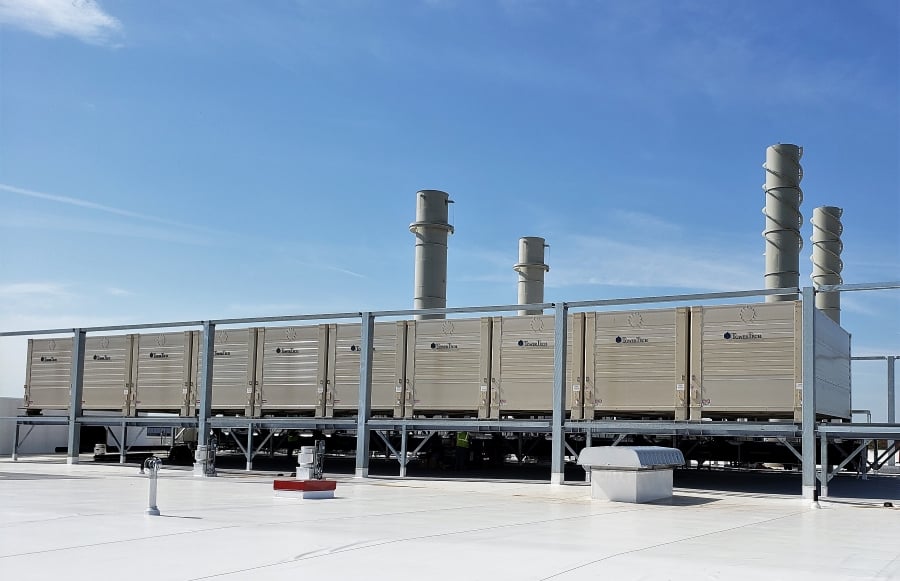
Tower Tech is the leader for applications where redundancy and energy efficiency are paramount. Our cooling towers can remain up-and-running even if a fan or other modular component goes down. When is comes to flexibility Tower Tech towers provide maximum utility to power plants and utilities providers.
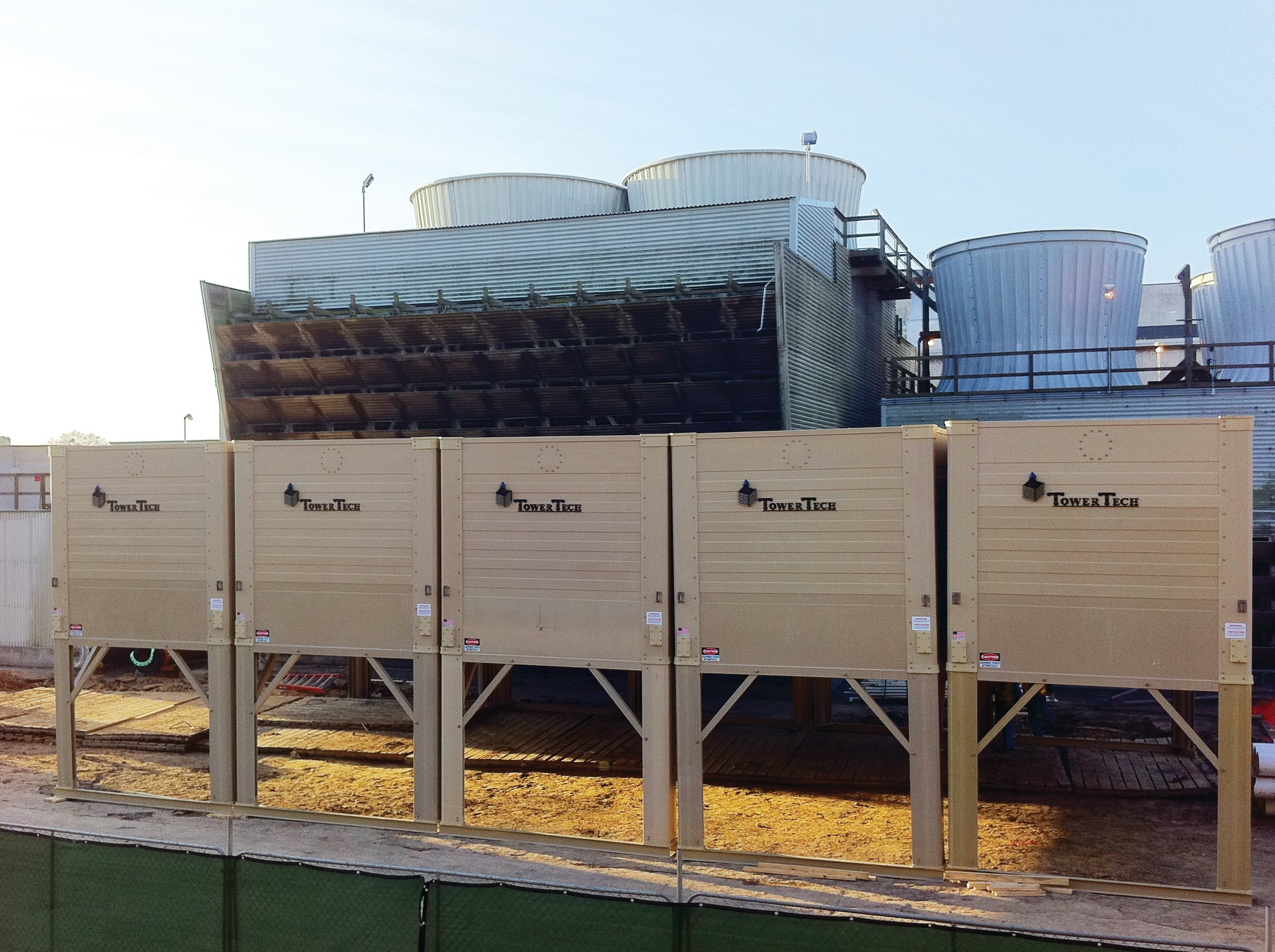
Tower Tech is the leader for applications that are seasonal or necessitate a short term cooling tower solution. Our cooling towers are modular and because they are factory built they can be easily transported and installed. Our technology can get your application or facility's cooling back on-line in the shortest amount of time.
Get the Show on the Road
Tower Tech created our BabyTech demo tower to showcase our tower's functionality as well as their modularity. The BabyTech is a fully functioning mini-tower that is 75% smaller than our standard 4-fan tower.